
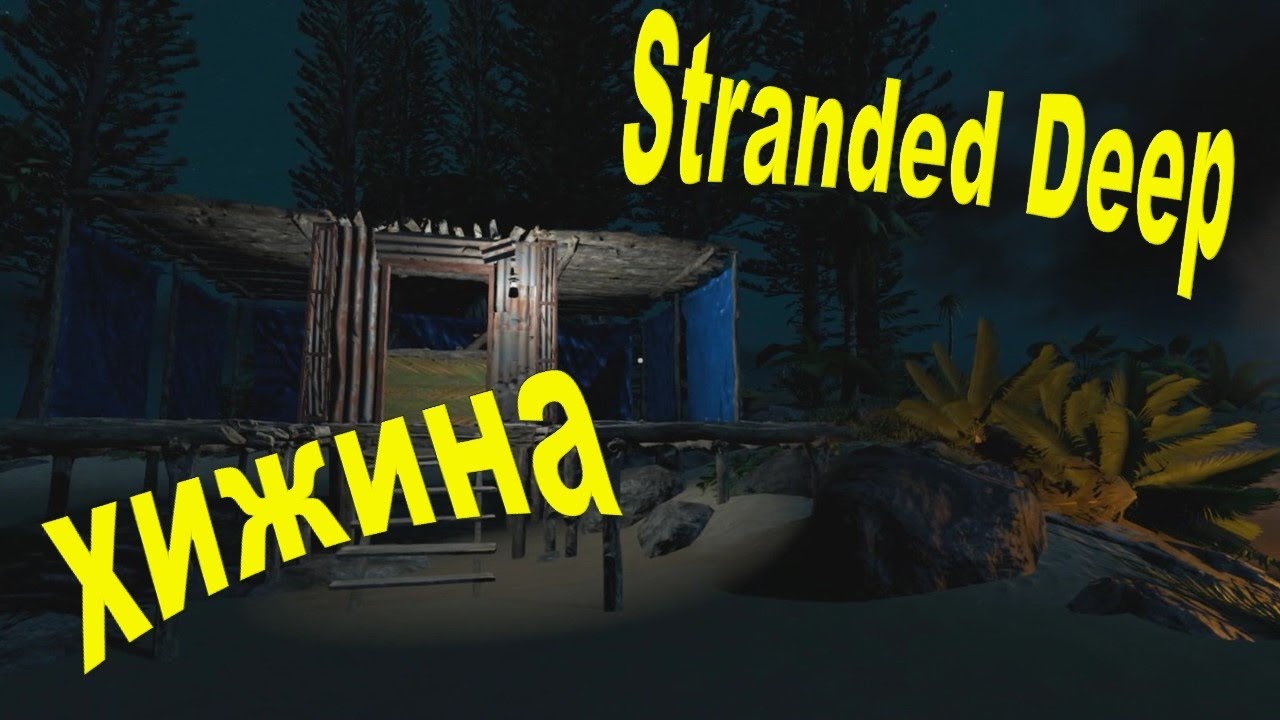
Identifying these adaptations and incorporating them into future iterations of this model will further reveal the factors driving GE in sea turtles.Ĭetaceans have long been considered biologically adapted to suffer no adverse effects from diving-related tissue gas tension. This likely means that turtles have additional behavioral, anatomical, and/or physiologic adaptions that serve to reduce the probability of GE but were not incorporated in this model. This baseline model also indicates that even during routine diving behavior, sea turtles are at high risk of GE. Our model suggests that cardiac output likely plays a significant role in the modulation of GE, especially in the deeper diving leatherback turtles. Finally, we varied body-condition and cardiac output within the model to see how these factors affected the risk of GE. Next, we applied this model to data collected from free-roaming individuals of the three study species. To parameterize the sea turtle model, we used values gleaned from previously published literature and 22 necropsies. This model was adapted from a published model for estimating gas dynamics in marine mammals and penguins. To better understand why sea turtles develop GE, we built a mathematical model to estimate partial pressures of N 2 (PN 2), O 2 (PO 2), and CO 2 (PCO 2) in the major body-compartments of diving loggerheads (Caretta caretta), leatherbacks (Dermochelys coriacea), and green turtles (Chelonia mydas). Sea turtles, like other air-breathing diving vertebrates, commonly experience significant gas embolism (GE) when incidentally caught at depth in fishing gear and brought to the surface. These studies enabled research on free-ranging common bottlenose dolphins in Sarasota Bay, FL, and off Bermuda, and have included work on the metabolic and cardiorespiratory physiology of both shallow- and deep-diving dolphins and have been integral to better understand how cetaceans can dive to extreme depths, for long durations. Studies on cardiorespiratory function have been integral to the development of this hypothesis, with work initially being conducted on excised tissues and cadavers, followed by studies on anesthetized animals or trained animals under human care. This has led to the selective gas exchange hypothesis, which provides a mechanism how stress-induced behavioral change may cause failure of the normal physiology, which results in excessive uptake of N 2, and in extreme cases may cause formation of symptomatic gas emboli. Studies on behavior, anatomy and physiology of marine mammals have enhanced our understanding of the mechanisms that are thought to prevent excessive uptake of N 2.
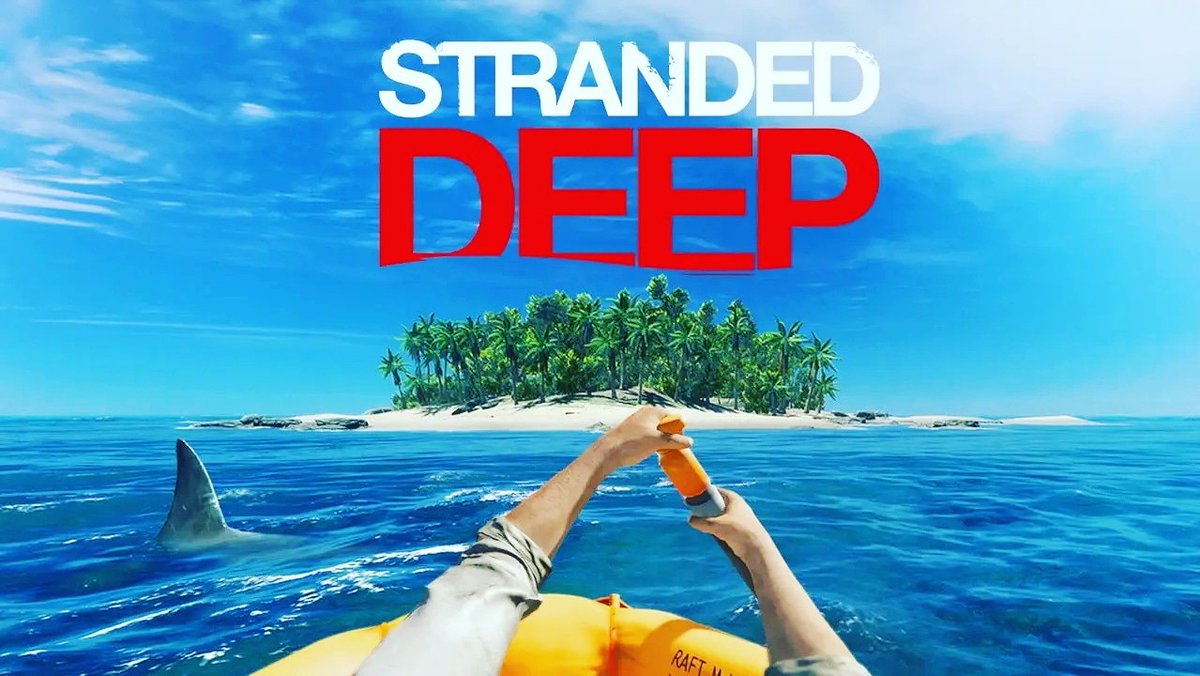
However, the dolphins appear to have physiological or behavioral mechanisms to avoid excessive blood N 2 levels, or may be more resistant to circulating bubbles through immunological/biochemical adaptations. Theoretical models, based on our current understanding of diving physiology in cetaceans, predict that the tissue and blood N 2 levels in the bottlenose dolphin ( Tursiops truncatus ) are at levels that would result in severe DCS symptoms in similar sized terrestrial mammals. Marine mammal management, and usual avoidance, of gas emboli and GEP, or the bends, became a topic of intense scientific interest after sonar-exposed, mass-stranded deep-diving whales were observed with gas bubbles. However, evidence that marine mammals experience gas bubbles during diving is growing, causing concern that these bubbles may cause gas emboli pathology (GEP) under unusual circumstances. Decompression theory has been mainly based on studies on terrestrial mammals, and may not translate well to marine mammals.
